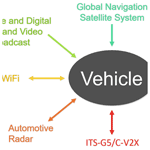
Human RF Electromagnetic Exposure to V2X-Communication
Lisa-Marie Schilling
Christian Bornkessel
Matthias A. Hein
In the era of automated and connected driving, more and more cars will be equipped with wireless transmission technologies such as mobile communications 4G (LTE) and 5G, WiFi, Bluetooth, and V2X. For the technical implementation of V2X-communications, different standards like cellular-V2X from the cooperation 3rd Generation Partnership Project and ITS-G5, based on the WiFi standard 802.11p from the Institute of Electrical and Electronics Engineers, are under consideration. The electromagnetic environment of cars and the corresponding exposure of the general public to wireless emission will be significantly influenced by new radio technologies. Under all circumstances, it must be ensured that the exposure of the electromagnetic fields inside a car does not cause any harmful effects on humans. In order to quantitatively assess the resulting exposure, the generated exposure must be correctly recorded and evaluated according to their specific time-frequency spectra. This paper describes a new measurement procedure suitable for the V2X-standard ITS-G5 together with various exposure measurements performed in different cars with WiFi, Bluetooth and ITS-G5. In comparison of all wireless standards studied here, the V2X-service generated the highest electric field strengths inside a car, when a transmitting di-patch antenna was mounted on the windscreen inside the driver's cabin. The maximum fraction of the corresponding ICNIRP reference level amounted to 15.1 %. We conclude that the total exposure of wireless on-board automotive devices will be dominated by ITS-G5, if the transmitting antenna is located inside the passenger cabin. As V2X-communications will increasingly penetrate road traffic, the resulting exposure should be carefully monitored, in order not to exceed the corresponding reference levels for general public.
Modern radio technologies are increasingly being used in everyday life so that the relevance of personal exposure to radio frequency (RF) electromagnetic fields is rapidly rising. In the context of automated and connected driving, vehicles will be capable of communicating with each other, as well as with infrastructure and other road users. For the technical implementation of this vehicle-to-everything (V2X)-communication, different standards like cellular-V2X (C-V2X) from the cooperation 3rd Generation Partnership Project (3GPP, 2019) and the European standard for vehicular communications ITS-G5, based on the WiFi standard 802.11p (IEEE, 2010) from the Institute of Electrical and Electronics Engineers (IEEE), are under consideration.
In addition to the V2X-communication standards, there are further wireless systems such as audio and video broadcast, mobile radio, infotainment systems et cetera, that interact with the environment of cars and thus influence personal exposure of traffic participants inside cars (driver and passengers) as well as outside (road users). Figure 1 shows a categorization of the various wireless standards and services. All of these wireless technologies contribute to personal exposure and, as a consequence, suitable measurement and evaluation methods must be developed, in order to correctly determine the electromagnetic field exposure and assess this in view of the legal human safety limits for RF electromagnetic radiation. Since reliable data on exposure associated with the wireless automotive technologies are often lacking, it is essential that the introduction of new technologies is accompanied by scientifically exposure studies. There is a particular need for research into the development of suitable measurement and assessment methods for evaluating exposure in the vehicular environment (SSK, 2019). This study aims to contribute to filling this research gap.
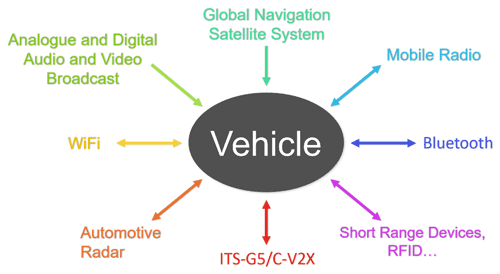
Figure 1Relevant wireless automotive technologies in the electromagnetic environment of road traffic; arrow directions indicate whether the wireless automotive technology is only received (technology → vehicle) or is received and sent (technology ↔ vehicle) by the vehicle.
The International Commission on Non-Ionizing Radiation Protection (ICNIRP) formulated reference levels for the electric and magnetic field strengths for frequencies above 2 GHz, namely 61 V m−1 and 0.16 A m−1, respectively, which must not be exceeded in areas accessible to the general public (ICNIRP, 1998). These ICNIRP limits still form the basis for exposure control legislation in most countries until today. This includes Germany, namely the law for protection against electromagnetic exposure: “Sechsundzwanzigste Verordnung zur Durchführung des Bundes-Immissionsschutzgesetzes (Verordnung über elektromagnetische Felder – 26. BImSchV)” (26. BImSchV, 2013).
The limits are based on the proven biological effect that the absorption of electromagnetic power primarily has a thermal effect on the human organism. The temperature increase is directly related to the temporal mean of the signal power, so that the reference limits are defined by root-mean-square (RMS) values. Accordingly, for digitally modulated signals with a noise-like fluctuating signal amplitude, time-averaged values must be measured instead of peak values. Moreover, the duty cycle must be accurately considered for pulse-shaped signals. The correct measurement of RMS values under all possible transmission modes thus represents the central challenge for evaluating the RF exposure.
The aim of this paper is to describe and verify experimental approaches to correctly determine the exposure to selected radio services inside a car. An overview of modern wireless automotive technologies is given in Sect. 2. Section 3 describes the ITS-G5 specific measurement and evaluation method which has been developed (Schilling et al., 2020), and measurements of the electric field strength generated through WiFi, Bluetooth and ITS-G5 in realistic automotive environments. Section 4 concludes the investigation of the exposure measurements.
A large variety of wireless transmission systems interacts with the traffic environment of modern cars and thus influences the human RF exposure. Mobile radio (GSM, UMTS, LTE, 5G) as well as Bluetooth and WiFi systems are especially used for communications, data transmission including over-the-air updates of software, and infotainment. Furthermore, receivers for various analogue and digital audio and video broadcast systems (AM, FM, DAB, DVB-T) and global navigation satellite systems (GNSS) are located inside the vehicle. Automotive radar systems, short range devices (SRD), and radio frequency identification (RFID) applications transmit relevant information to driver assistance systems, remote keyless entry or vehicle identification systems. In the future, the implementation of technologies such as ITS-G5 or C-V2X will also increase in view of connected and automated driving. An overview of the wireless automotive technologies is shown in Fig. 1, and their frequency ranges as well as their maximum permissible equivalent isotropically radiated power (EIRP) from possible on-board devices are summarised in Table 1. In case of mobile radio uplinks, Table 1 contains the standard-specific transmit power of user devices as this, and not the EIRP, is the standardised feature here. The values refer to European specifications and may slightly differ from other regional standards (e.g. USA or Asia-Pacific countries). We can draw the following conclusions from this data collection:
Table 1Overview of relevant wireless automotive technologies and their frequency range as well as maximum permissible mean EIRP (Schilling, 2019; BNetzA, 2020).
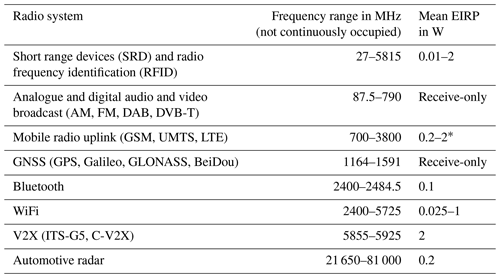
∗ Transmit Power.
The prospective electromagnetic environment of cars will be significantly influenced by V2X, since the corresponding user terminals are installed in the immediate vicinity of, or directly inside, the car. Every road user should be connected, so that on a long-term basis the technology is expected to be installed in all vehicles. The V2X devices can also transmit with a higher EIRP than other radio equipment such as LTE, WiFi or Bluetooth installed directly in the vehicle (Schilling, 2019). In Europe, V2X systems are allowed to transmit with a maximal EIRP of 2 W whereas, for example, LTE user equipment transmits at power levels remaining below 0.2 W. Table 1 indicates a maximal transmit power of 2 W for mobile radio uplinks, but only GSM devices exploit this limit. The EIRP of Bluetooth and WiFi operating at 2.4 GHz is even limited to 0.1 W. Automotive radar systems can transmit with an EIRP of up to 316 W, but this value denotes the maximum peak EIRP instead of a mean value. As automotive radar systems often transmit pulsed-shaped signals, the mean EIRP is far less (about 0.2 W; BNetzA, 2020). It should therefore be examined how the exposure of road users through the new V2X technologies changes in comparison to the already established communication systems in the car.
3.1 Development of measurement methods
The exposure through ITS-G5, WiFi and Bluetooth should be determined inside a car for a proper quantitative comparison. Therefore, the frequency selective measurement instrumentation SRM-3006 from Narda STS (Narda Safety Test Solutions, 2020) was used in connection with the corresponding isotropic antenna (type 3502/01, frequency range 420–6000 MHz), which represents an established setup for the exposure evaluation of different radio technologies (Wuschek, 2019). The community of electromagnetic compatibility (EMC) usually considers an expanded uncertainty (JCGM, 2008) which, for the case of the SRM-3006 in connection with a 1.5 m RF cable, is recommended as +1.9/−2.5 dB for uniaxial measurements in the frequency range 5–6 GHz (Narda Safety Test Solutions, 2020). For exposure measurements, this measurement uncertainty is typically extended to ±3 dB, in order to account for uncertainties during taking measurement samples.
An appropriate novel measurement and evaluation procedure to correctly record and assess exposure to the ITS-G5 wireless standard was previously developed and described in Schilling et al. (2020). In the time domain, the ITS-G5 signal structure has a pulsed rectangular shape with a significant crest factor (CF) of up to 7 dB on the pulse roofs, as illustrated in Figs. 2 and 3, respectively.
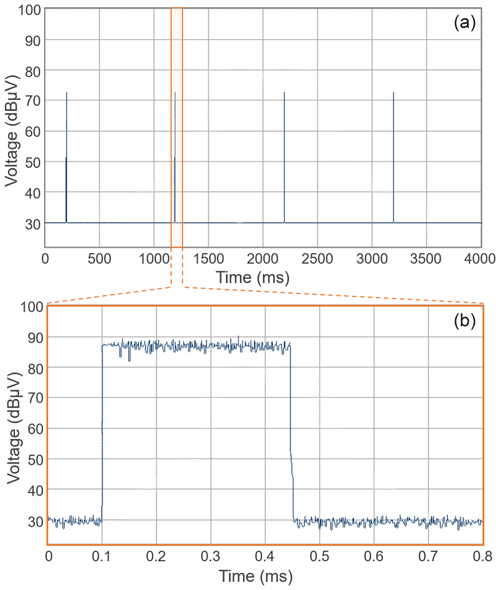
Figure 2Time domain sections of the ITS-G5 signal at 5.9 GHz. The period of the pulse sequence shown on the top is one second and the rectangular pulse shown on the bottom is 345 µs long.
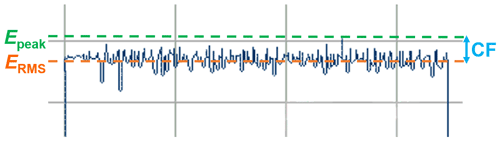
Figure 3Detailed view of the ITS-G5 pulse roof from Fig. 1. The crest factor of ITS-G5 (CF, ratio of peak value Epeak to RMS value ERMS) can be up to 7 dB.
The CF is defined as the ratio of peak value Epeak to RMS value ERMS in linear units:
The challenge of measuring the ITS-G5 signal is that the pulses should not coincide with the delay time of the measurement device but are recorded completely. Delay times occur due to 20 ms long pauses, partly caused by switching between the three orthogonal measuring axes during an isotropic measurement, and due to pauses in the digital signal processing (Keller, 2009). The setting Level Recorder was identified as the most suitable operating mode for the SRM-3006 for ITS-G5 pulses, as it can record a signal seamlessly at a fixed centre frequency and resolution bandwidth. Delay times caused by switching can be avoided by measuring the electric field strength in the three orthogonal axes Ex, Ey, Ez individually and combining them to an isotropic value Eiso via quadratic summation subsequently:
Furthermore, it is important to ensure that the signal level is neither over- nor underestimated, depending on the CF and the duty cycle of the signal. An RMS detector mode is not available in the SRM-3006, but the signal can be smoothened with a video filter whose averaging time should be of the order of the magnitude of the pulse duration (Keller, 2011). The recorded RMS field value of the pulse roof can then be extrapolated to any duty cycle by a suitable correction factor. To determine the ultimate exposure, the maximum possible duty cycle is calculated based on the standard-specific limit for pulse duration τmax and the minimum period Tmin, leading to the correction factor Kmax (Schilling et al., 2020):
According to the predicted channel load in the ITS-G5 control channel around 5.9 GHz, the message type “cooperative awareness message” (CAM) will use more than 90 % of the channel capacity (C2C, 2014). The CAM is sent periodically by each road user, to provide information about their location. Its standard minimum period Tmin is 100 ms. The maximum pulse duration τmax of 4160 µs can be derived from the physical layer specifications of the standard, resulting in a correction factor for the worst-case duty cycle Kmax = −13.8 dB. Other ITS-G5 message types such as “decentralized environmental notification message” or “signal phase and timing” will not influence the personal exposure as strongly as CAMs, because they will contribute to a small amount of the transmission load only. Therefore, the message type CAM was in the focus of our investigations of the exposure through ITS-G5.
The WiFi and Bluetooth signals were recorded with the same principle as ITS-G5, where the correction factors to extrapolate the duty cycle to maximum load were adjusted according to the corresponding standards. It is possible to record the beacon pulses from a WiFi access point with a maximum possible duty cycle for the whole data signal of 90 % (Schmid et al., 2005). For an extrapolation of the measured field strength from one beacon pulse to duty cycle = 90 %, the correction factor Kmax amounts to −0.5 dB. Bluetooth also has a pulsed signal structure in the time domain whose maximum duty cycle is considered in the processing of the measured values by the correction factor Kmax = −1.1 dB (max. load: τmax ≈ 2.9 ms; Tmin = 3.75 ms) (Hofmann et al., 2018).
3.2 Measurement setups
Several user equipment devices were employed for the measurements to assess the exposure at typical locations in passenger cars. The electric field strengths generated by an originally integrated WiFi and Bluetooth system, an external Bluetooth hands-free system, and two ITS-G5 variants were measured in different cars which are summarized in Table 2. For the ITS-G5 measurements inside the car, the on-board-unit (OBU) MK5 from Cohda Wireless (Cohda Wireless, 2020) was operated using the magnet-mounted antenna MGW-303 (Mobile Mark, 2020) on the car roof, and, alternatively, a di-patch antenna developed in house (Singh et al., 2017, 2019) mounted on the upper corner of the windscreen in front of the driver's seat, each fed with an EIRP of 2 W. These exemplary antenna placements were chosen as they reflect potential realistic installation locations for upcoming automotive V2X-terminals.
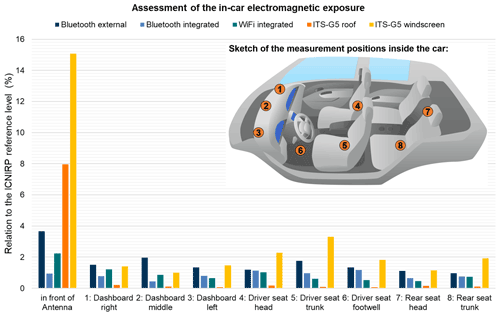
Figure 4Assessment of the electromagnetic exposure to different radio-based devices at nine measurement positions inside cars evaluated in terms of the ICNIRP reference level.
The OBU was configured to send CAMs as part of a road traffic simulation, because this message type will probably dominate the ITS-G5 channel load and, consequently, the personal exposure regarding V2X-communications. In order to systematically compare the results, measurement positions were predefined at several locations inside the cars as illustrated in the upper right part of Fig. 4. An additional measurement was made in direct physical contact with the respective transmitting antenna and the isotropic probe, where the diameter of the probe was 12 cm.
3.3 Results and discussion
Figure 4 summarises the evaluation results from the measurements with the selected radio systems at the nine different measurement positions. The data were scaled to the fraction of the ICNIRP reference level of 61 V m−1 for each measurement point. Table 3 contains the highest measured values for each device derived from the in-car measurements. The measurement uncertainty was determined to be ±3 dB but was not added to the presented results.
Table 3Maximum percentage of the ICNIRP reference level for each device evaluated during the in-car measurements.
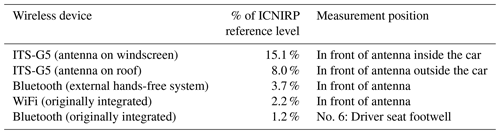
It was found that the mounting position of the transmitting antennas has a strong influence on the prevailing field strengths. The ITS-G5 variant which contains the antenna on the inner side of the windscreen, causes significantly higher exposure values (factor 3 on average) than the other devices. ITS-G5 transmits at a higher power than Bluetooth and WiFi as outlined in Table 1. The field strength of the car roof antenna is strongly attenuated by the car body and windows. The field strengths of the Bluetooth and WiFi signals were in a comparable range, with the external Bluetooth hands-free system tending to generate higher emissions (factor 1.9 on average) than the internally installed infotainment systems. The transmitting antenna of the external hands-free kit was located directly in the passenger cabin and covered just by a thin plastic radome. The location of the originally integrated WiFi and Bluetooth systems inside the test cars was identified to be in front of the centre control elements for radio and air conditioning, as the maximum electric field strength was found there.
In summary, the maximum fraction of the ICNIRP reference level of 15.1 % was determined inside a car with the ITS-G5 antenna mounted on the windscreen. We conclude that the total exposure to wireless on-board automotive devices will be dominated by ITS-G5, if the transmitting antenna is located inside the passenger cabin. In order to minimise exposure of passengers, transmitting antennas should not be installed directly in the car interior.
As only nine locations inside the car were used as measurement positions, the possible maximum of the electric field strength in the entire interior cabin might have been missed. Although the highest field strengths mostly occurred in the immediate vicinity of the transmitting antennas, resonant field enhancements could hypothetically arise in different car interiors, at different positions for different wireless technologies. Since these phenomena depend on their peculiar geometric and electrical environments, the quantitative results always reflect, and are limited to, the measurement scenario under consideration. During the measurement campaigns, however, no limit values were found to be exceeded with respect to the ICNIRP reference levels for the general public. Further studies to assess the possibility and potential strengths of resonances are in progress.
With the introduction of wireless automotive technologies, there is an urgent need for scientific research to evaluate the corresponding human RF exposure in the vicinity of connected vehicles. Therefore, appropriate measurement and assessment methods should be developed and scientific studies should be conducted to fill this current gap in exposure research to electromagnetic fields. Thus, in the presented study, the exposure to RF radio systems inside cars was recorded and evaluated according to the ICNIRP reference values. A newly developed wireless standard-specific measurement and evaluation method for ITS-G5 to record exposures of the V2X-communication technology was discussed in addition to WiFi and Bluetooth. With the aid of a correction factor for taking into account standard-specific duty cycles, the RMS electric field strengths of the various radio systems inside the car can be determined and correctly evaluated in relation to the reference levels.
In comparison of all wireless standards studied, ITS-G5 generated the highest electric field strength. As V2X-communications will increasingly penetrate road traffic, the resulting exposure contributions should be carefully monitored, in order not to exceed the corresponding exposure limits. In addition to the exposure of passengers inside a car, the question of the exposure of exterior pedestrians in the traffic environment also presents an important research topic, to be investigated next. Thus, not only are radio systems installed inside vehicles, but also more and more road side units for V2X-communication or small cells for mobile radio coverage are installed in the immediate vicinity of the general public. Therefore, the verification of exposure levels with reliable and practicable measurement methods, as presented in this paper, will be indispensable in the future.
The data were generated as part of the master thesis “Erfassung der elektromagnetischen Umwelt beim KFZ im Hinblick auf automatisiertes und vernetztes Fahren” from Lisa-Marie Schilling at the Technische Universität and can be made available upon request.
LMS worked on the research, the development of the exposure measurement and assessment methods and performed the measurements under the supervision of CB and MAH. LMS prepared the manuscript as main author with feedback from CB and MAH.
The contact author has declared that none of the authors has any competing interests.
Publisher’s note: Copernicus Publications remains neutral with regard to jurisdictional claims in published maps and institutional affiliations.
This article is part of the special issue “Kleinheubacher Berichte 2020”.
This paper was edited by Ludger Klinkenbusch and Madhu Chandra, and reviewed by Bhavinkumar Patel and Emre Colak.
26. BImSchV: Sechsundzwanzigste Verordnung zur Durchführung des Bundes-Immissionsschutzgesetzes (Verordnung über elektromagnetische Felder – 26. BImSchV), BGBl. Jg. 2013 Teil I Nr. 50, Federal law, Germany, 2013.
3GPP: ETSI TS 136 300 V14.11.0, LTE; Evolved Universal Terrestrial Radio Access (E-UTRA) and Evolved Universal Terrestrial Radio Access Network (E-UTRAN); Overall description; Stage 2 (3GPP TS 36.300 version 14.11.0 Release 14), Tech. Spec., https://www.etsi.org/deliver/etsi_ts/136300_136399/136300/14.11.00_60/ts_136300v141100p.pdf (last access: 14 September 2022), 2019.
Bundesnetzagentur (BNetzA): Vfg. 53/2020, Allgemeinzuteilung von Frequenzen für Verkehrs- und Verkehrstelematikgeräte, https://www.bundesnetzagentur.de/SharedDocs/Downloads/DE/Sachgebiete/Telekommunikation/Unternehmen_Institutionen/Frequenzen/Allgemeinzuteilungen/Verkehrsanwendungen/vfg532020.pdf;jsessionid=D25C2F1541BDEBCF0BEAFFCFAC3BBEAE?__blob=publicationFile&v=3 (last access: 14 September 2022), 2020.
Car 2 Car Communication Consortium (C2C): ETSI ITS G5 Channel Usage, Position Paper, Car 2 Car Communication Consortium, https://www.car-2-car.org/fileadmin/documents/General_Documents/C2CCC_TR_2049_Channel_Usage.pdf (last access: 14 September 2022), 2014.
Cohda Wireless: MK5 OBU Specification, Datasheet, https://www.cohdawireless.com/wp-content/uploads/2018/08/CW_Product-Brief-sheet-MK5-OBU.pdf (last access: 13 September 2022), 2020.
Hofmann, W., Bornkessel, C., and Hein, M. A.: Entwicklung eines Immissionsmessverfahrens für Funkdienste mit Frequenzsprungverfahren, emv, Internationale Fachmesse und Kongress für Elektromagnetische Verträglichkeit, 20–22 February 2018, Düsseldorf, Germany, https://doi.org/10.15488/4379, 2018.
ICNIRP: Guidelines for Limiting Exposure to Time-Varying Electric, Magnetic and Electromagnetic Fields (100 kHz to 300 GHz), Health Phys., 74, 494–522, 1998.
IEEE: Standard 802.11p-2010 Part11 Amendment 6, IEEE Standard, IEEE Computer Society, 2010.
JCGM: Evaluation of measurement data – Guide to the expression of uncertainty in measurement, JCGM 100:2008 (GUM 1995 with minor corrections), 1st edn., https://www.bipm.org/documents/20126/2071204/JCGM_100_2008_E.pdf/cb0ef43f-baa5-11cf-3f85-4dcd86f77bd6 (last access: 14 September 2022), 2008.
Keller, H.: Principles of the Selective Radiation Meter SRM-3006, Narda Safety Test Solutions, Application Note, 2009.
Keller, H.: Bewertung rauschartiger Impulse moderner Kommunikationssysteme, Jahrestagung des Fachverbandes für Strahlenschutz e.V., 19–21 September 2011, Dortmund, Germany, 209–223, 2011.
Mobile Mark: MGW-303 Series Antennas, Datasheet, https://www.mobilemark.com/download/2033/gps-gnss-multiband/7329/smw-303-and-mgw-303-spec-sheet.pdf (last access: 13 September 2022), 2020.
Narda Safety Test Solutions: Operating Manual SRM-3006, https://www.narda-sts.com/de/produkte/selektiv-emf/srm-3006-field-strength-analyzer/pd/pdfs/23165/eID/ (last access: 13 September 2022), 2020.
Schilling, L.-M.: Erfassung der elektromagnetischen Umwelt beim KFZ im Hinblick auf automatisiertes und vernetztes Fahren, Master thesis, Technische Universität Ilmenau, Ilmenau, unpublished, 2019.
Schilling, L.-M., Bornkessel, C., and Hein, M. A.: Entwicklung und Verifikation eines Mess- und Bewertungsverfahrens der elektromagnetischen Immission durch ITS-G5, emv, Internationale Fachmesse und Kongress für Elektromagnetische Verträglichkeit, 17–19 March 2020, Köln, Germany, 457–464, https://doi.org/10.15488/10058, 2020.
Schmid, G., Lager, D., Preiner, P., Überbacher, R., Neubauer, G., and Cecil, S.: Bestimmung der Exposition bei Verwendung kabelloser Übermittlungsverfahren in Haushalt und Büro, Schriftenreihe Reaktorsicherheit und Strahlenschutz, Publication series BMU-2005-669, Germany, Bundesministerium für Umwelt, Naturschutz und Reaktorsicherheit, ISSN 1612-6386, 2005.
Singh, J., Stephan, R., and Hein, M. A.: Strahlungselement, Deutsches Patent- und Markenamt, Appl. 10 2017 011 225.8, 2017.
Singh, J., Stephan, R., and Hein, M. A.: Low-Profile Wideband Differentially Fed Di-Patch Antenna Closely Above Metallic Ground, IEEE Antenn. Wirel. Pr., 18, 976–980, https://doi.org/10.1109/LAWP.2019.2906825, 2019.
SSK: Electromagnetic fields in vehicles generated by radio applications, inductive charging and electromobility, Recommendation of the German Commission on Radiological Protection with scientific background, Germany, Strahlenschutzkommission, https://www.ssk.de/SharedDocs/Beratungsergebnisse_PDF/2019/2019-06-27EMFAuto_e.pdf?__blob=publicationFile (last access: 13 September 2022), 2019.
Wuschek, M.: Measuring RF Electromagnetic Fields at Mobile Communications Base Station and Broadcast Transmitter Sites, Narda Safety Test Solutions, Issue 1, Germany, ISBN 978-3-00-061912-0, 2019.